I fell in love with the beauty of proteins
Meet Sheena Radford from the University of Leeds
October 13, 2022
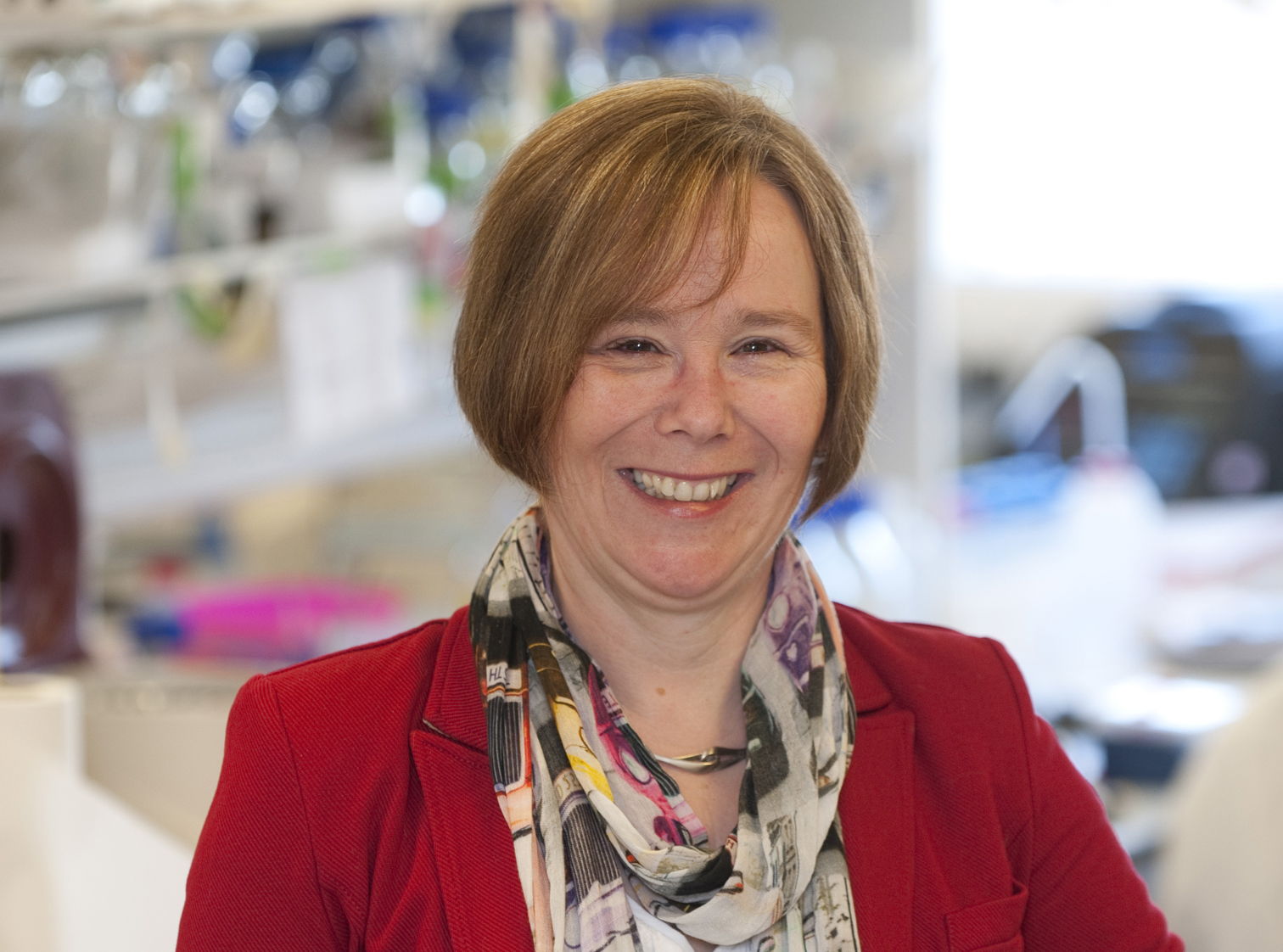
This blog post is part of VIB Neuroscience in the spotlight.
On the last day of the 2022 Protein Aggregation Meeting in Leuven, Professor Sheena Radford gave a captivating lecture on the dynamics and kinetics of amyloid fibril growth and the potential relevance of amyloid polymorphs in disease pathology. We spoke to Sheena about her latest insights into amyloid fibril formation and the challenges that lie ahead for elucidating how amyloid fibril formation contributes to human disease.
Sheena Radford is Astbury Professor of Biophysics at the Astbury Centre for Structural Molecular Biology at the University of Leeds (UK) and Royal Society Research Professor. Her research is focused on fundamental structural molecular biology, specifically the measurement of the conformational dynamics of proteins and the elucidation of the role that these motions play in protein folding and misfolding in health and disease. Sheena was invited to give a lecture at the Protein Aggregation Meeting in Leuven, organized by Joost Schymkowitz, Frederic Rousseau, Hannah Wilkinson, and Béla Z Schmidt from the Switch lab. After her lecture, we spoke to her about the wonderful world of protein aggregation.
A biochemist by training, Sheena fell in love with the beauty of the structures of proteins as soon as she saw them as an undergraduate student. “I immediately wanted to know how such beautiful molecules work. During my PhD on enzyme function at the University of Cambridge, I realized that the malleability of proteins and their internal motions are critical for their function, and this was what interested me the most. Right from the beginning, this kind of protein dynamics and its impact on function, was utterly fascinating. Later on, as a postdoc and research fellow in Oxford, I began to ponder how the beautiful structures of proteins can be built from just 20 different types of amino acid: the so-called protein folding problem and what happens when folding goes awry”.
Her interest in protein folding commenced when a postdoc at the University of Oxford in the later 1980s where, working with the late Sir Chris Dobson, new methods and approaches were developed that were able to show how the enzyme lysozyme from hen eggs and from humans is able to fold to its correct, enzymatically active structure. A telephone call from Sir Mark Pepys from the Hammersmith Hospital in London later opened up new horizons that built on this fundamental discovery research. “Protein micro-sequencing had come on board in those days. Prof Pepys had collated frozen samples of amyloid plaques taken from his patients with amyloid disease. Upon sequencing, some of these plaques turned out to consist of mutated human lysozyme”, explains Sheena Radford. “Since we were working on the folding dynamics of lysozyme, Prof Pepys asked us whether we could explain how single point variants in human lysozyme made a misfolded protein and led to disease. That question started my research career in amyloidosis. And the rest is history.”
To date close to 50 human proteins or peptides have been found to form amyloid that is associated with human disease. The hallmark of amyloid disease is the aggregation of proteins into amyloid fibrils and their deposition into extracellular plaques and intracellular inclusions. The most prevalent amyloid disorders, affecting millions of people worldwide, include Alzheimer’s disease, Parkinson’s disease, and amyotrophic lateral sclerosis, all neurodegenerative disorders. Amyloidosis can also involve systemic or localized deposits of amyloid, such as in dialysis-related amyloidosis in which b2-microglobulin forms amyloid in joints, or type 2 diabetes where we find deposits of amylin (also known as islet-associated polypeptide or IAPP) in pancreatic cells.
Despite the first observation of amyloid deposits nearly four centuries ago, it has been a long wait to see the structures of amyloid fibrils associated with devastating conditions such as Alzheimer’s disease and Parkinson’s disease in atomic detail.
“We know since the early work of Sandy Geddes, Bill Aiken, and colleagues in 1968, done here in Astbury’s Department of Biophysics in Leeds (who worked on a functional amyloid from the lacewing fly), that amyloid fibrils share a common underlying architecture in which the β-strands within each protofilament align perpendicular to the long axis of the fibril. This structure, they coined a ‘cross-β fold’, is marked by a characteristic ~4.7–4.8 Å repeat running down the fibril axis. This fold involves extensive mainchain hydrogen bonding between adjacent b-strands within the stacked layers. Although amyloid folds look the same at first sight, in detail, they are all different, as we now know.” Recent discoveries about the structure of amyloid fibrils have shifted our understanding of these fibrils look completely. They are much more interesting and much more complex than a simple set of repeating structural elements. They have a complex architecture, including twisting filaments and surfaces covered by disordered domains.
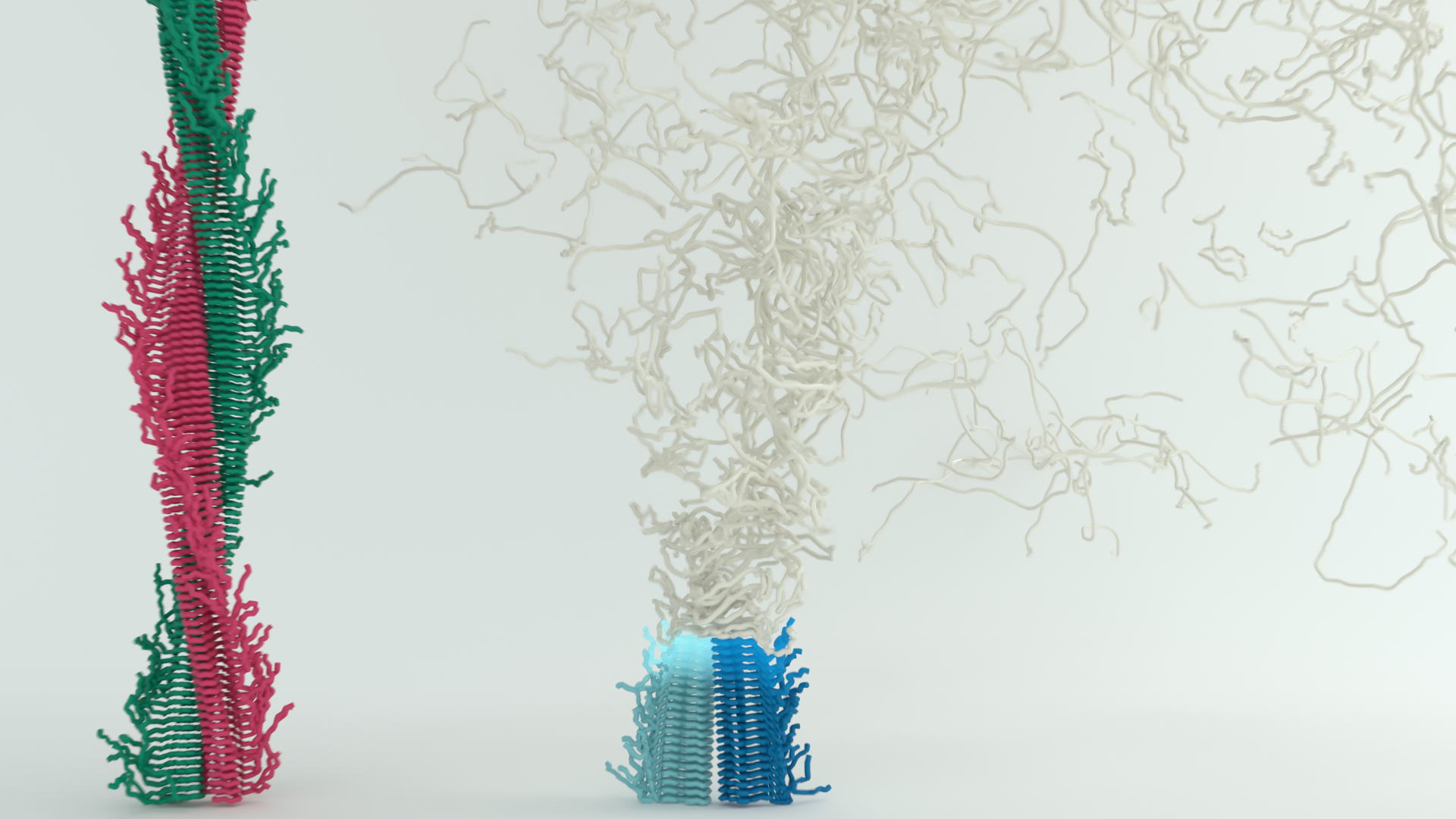
In the past five years, fibril structures have been determined in near-atomic detail, thanks to developments in methods to create fibrils in vitro, to purify them from ex vivo material, as well as breakthroughs in solid-state NMR spectroscopy, cryo-electron microscopy and the enormous increase in computational capacity. “With the newest cryo-electron microscopes, we are now getting single particle images of amyloid fibrils with a resolution of 2.2 Å. All different orientations of these helically twisting fibrils can be seen in the same sample and a highly detailed 3D image can be constructed computationally. With the current resolution, we can actually see bound water molecules. We are entering a new era of integrative structural biology that allows us to ‘see’ the structure of amyloid fibrils on multiple scales, from individual subunits and how they form fibrils, to the cellular consequences of fibril deposition into plaques and tangles. This has led to completely new insights.”
Sheena Radford gives a few examples of such new understandings: “We have known for centuries that amyloid filaments are extremely stable, both mechanically and thermodynamically, far exceeding the stability of the native fold of functional proteins. Insights over the last decade show that, despite their stability, fibrils are nonetheless dynamic structures that have a massive effect on their own propagation and on the health of cells. On the one hand, fibril surfaces act as potential sites for secondary nucleation, which is the catalytic growth of new fibrils from their surfaces. On the other hand, they are able to dissociate releasing oligomers and monomers from their ends.”
“We also see that the same protein sequence can form different amyloid structures. For example, a-synuclein, a culprit protein of Parkinson’s disease, can form at least two distinct polymorphs in the brain and more than 30 amyloid structures when made in a test tube. For Tau, different neurodegenerative diseases result in fibrils with distinct, disease-specific structures. The driver behind this fibril diversity is still under investigation. The intrinsic properties of the polypeptide sequence play a role, of course, i.e., variations in the sequence of the protein can lead to different amyloid folds and different folding kinetics. But there is also the environment, the presence or absence of post-translational modifications, and the interactions with other biomolecules, cellular components, and cofactors, all of which can play a role in driving amyloid assembly into a particular structure.”
“Another remarkable characteristic of amyloid fibrils determined recently is the high structural variability that can be created by the association of subunits with the same cross-b fold. And, perhaps most fascinating of all, the discovery that most, if not all, amyloid fibrils contain locally disordered regions that map both to the termini, as well as to internal segments of the proteins. The importance of these regions in amyloid formation is only now becoming clear. Despite the difficulties in characterizing the structures of these dynamic regions, they should not be overlooked since they have an impact on the kinetics of fibril growth and they interact with various cellular components, from cofactors and chaperones to membranes. These dynamic regions are also thought to be key players in causing cellular dysfunction and disease.”
“The contribution of our research group to the field is really in ‘topping and tailing’ the whole amyloid area from a structural mechanistic viewpoint. Half of my lab works on the initiating stages of amyloid fibril formation: what makes a protein amyloidogenic? What kicks off that reaction? What distinguishes a good fold from a bad fold? How does a single-point mutation make a protein become amyloidogenic? What are the kinetics and dynamics behind the folding process? If we know all of this, how can we inhibit or control amyloid formation?”
“Another area that we contributed to is that amyloid fibrils have ‘fuzzy coats’ or dynamic flanking regions, as mentioned earlier. Only a small proportion of the polypeptide chain sometimes contributes to the cross-b fold. The amyloid part of a-synuclein in disease, for example, is only formed by about 50 residues of the 140 amino acids in the whole protein. As a result, every 4.8 Å, you have 90 residues of very dynamic chains on the fibril outside. They form a unique biological environment. We are really interested in understanding how the dynamic regions, with their different architectures, contribute to the different polymorphs, the kinetics of filament growth, and their potential roles in health and disease”.
From the perspective of Sheena Radford, one has to look at amyloidosis from two perspectives. One is from a fundamental viewpoint: understanding how the amino acid sequence can encode this array of beautiful filament architectures that are created in the laboratory. And then on top of that, there is the question of how biology changes that repertoire of sequences and leads to amyloid folds in vivo.
“If you don't understand the basic structural mechanisms, you can't understand how biology tailors those mechanisms,” says Radford with insistence.
And then there is that third question: how to intervene with drugs to control amyloid formation in vivo. “This is an immensely challenging mission. There is first of all the diverse number of aggregation pathways and the multi-conformational nature of the related polypeptides. But also, the experimental pipelines for the identification of disease-modifying molecules are underdeveloped. And there also remains the fundamental question of whether it is better to slow down or speed up aggregation? If slowing down the aggregation process, which we all think might be the way to go, is associated with an accumulation of toxic intermediates, this could turn out to make things worse, not better. Maybe for some diseases, speeding up the assembly to less toxic amyloid fibrils could be beneficial. For other diseases, inhibiting the process could be the way to go. We have to develop a molecular toolkit that enables us to control the rates of aggregation, to know whether speeding up or slowing down is good or bad in specific biological processes and diseases.”
“The amyloid field has a long and sometimes agonizing history”, concludes Sheena, “and things have become even more complex in recent years. On the one hand, rather than a generic and simple amyloid fold, there is a remarkable diversity of amyloid structures, each of which may uniquely contribute to disease and cellular dysfunction. Thus, we have to look far beyond the cross-b fold. On the other hand, we are generating new insights into the fundamentals of the aggregation mechanisms, their kinetics and dynamics, and the structure of amyloid fibrils themselves at an amazing speed. We have an array of new strategies and technologies at our disposal. The amyloid field is currently full of hope and excitement. The field is buoyant and the hope for new understanding and new routes to therapy has never been closer!”
Learn more
If you want to dive deeper into brain health and disease, explore some of our resources, like our Alzheimer's facts series, or keep an eye out for interesting news and events where our researchers share new insights. We also have plenty of open positions for people eager to join us on our multi-faceted mission to unravel the mysteries of our brain.
Visit the webpage to find out more about VIB Neuroscience in the spotlight.