(Re)building life: The past, present, and future of synthetic biology
The field of synthetic biology has seen remarkable developments in the last decade or two
November 20, 2024
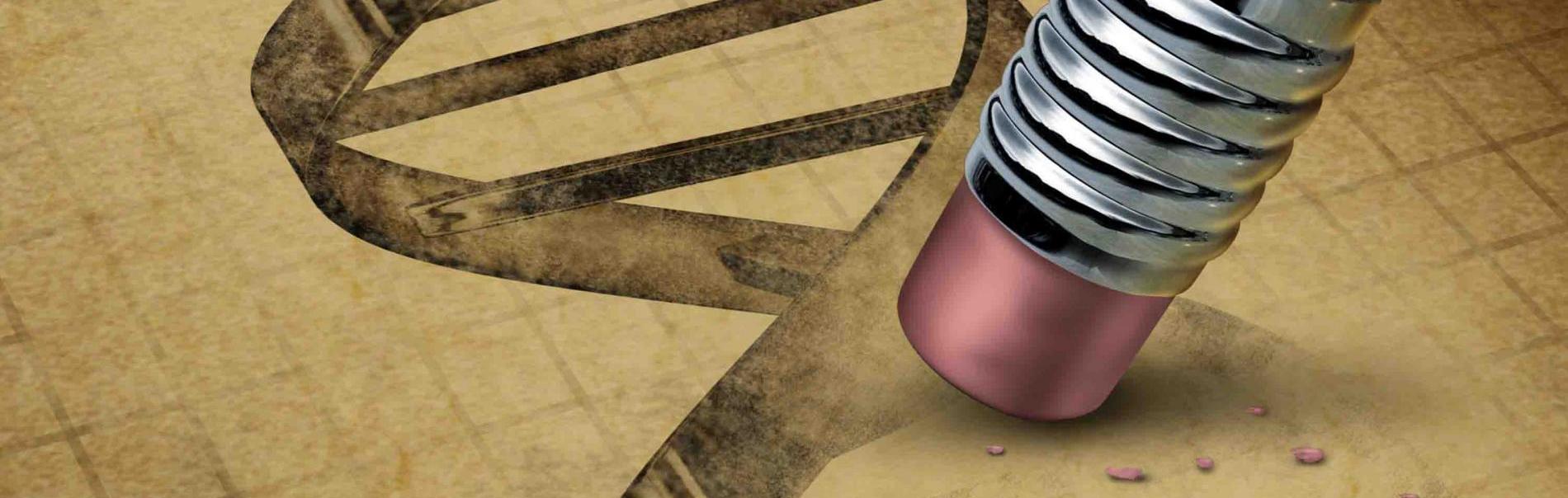
If we understand living systems as wholes constructed from parts, can we design new parts or redesign existing ones to build living systems and components according to desired specifications?
That is the promise of synthetic biology: (re)design biological building blocks and put them together into a system of your choice. Of course, life is more complicated than that. And yet, the field of synthetic biology has seen remarkable developments in the last decade or two.
In 2000, two papers in the journal Nature introduced the genetic toggle switch and the repressilator. These represent the first genetic circuits purposefully designed by humans. The toggle switch can turn target gene expression on and off while the repressilator oscillates like a circadian rhythm clock, periodically inducing the synthesis of GFP in individual cells.
The big leap, though, arrived in 2012 with the paper that introduced CRISPR/Cas to the world as a new generation of gene editing tool. CRISPR/Cas revolutionized genetic engineering by enabling precise, accurate, and efficient genetic alterations. In the wake of this discovery, synthetic biology flourished. Synthetic minimal cells and sophisticated gene circuits pushed the boundaries of what engineered cells can be – from sensors to cancer fighters. Gene drives emerged as a powerful, yet controversial, tool for controlling populations of disease vectors like mosquitoes. Synthetic biology's role in medicine led to the advent of mRNA vaccines and CAR-T cell therapies. AI and machine learning accelerated the design and optimization of synthetic biology experiments, while DNA data storage advanced toward becoming a practical solution for future data needs.
In 2020, both Emmanuelle Marie Charpentier and Jennifer Doudna received the Nobel Prize in Chemistry for their work on genome editing with CRISPR; the first time in history that the Nobel Prize was won by two women.
Let’s take a look at the present and future of synthetic biology, and what better way to do that than by considering the work of Anastassia Vorobieva (VIB-VUB Center for Structural Biology) and Sibylle Vonesch (VIB-KU Leuven Center for Microbiology), who are part of the organizing committee for our coming Next-Generation Synthetic Biology (5th edition) conference?
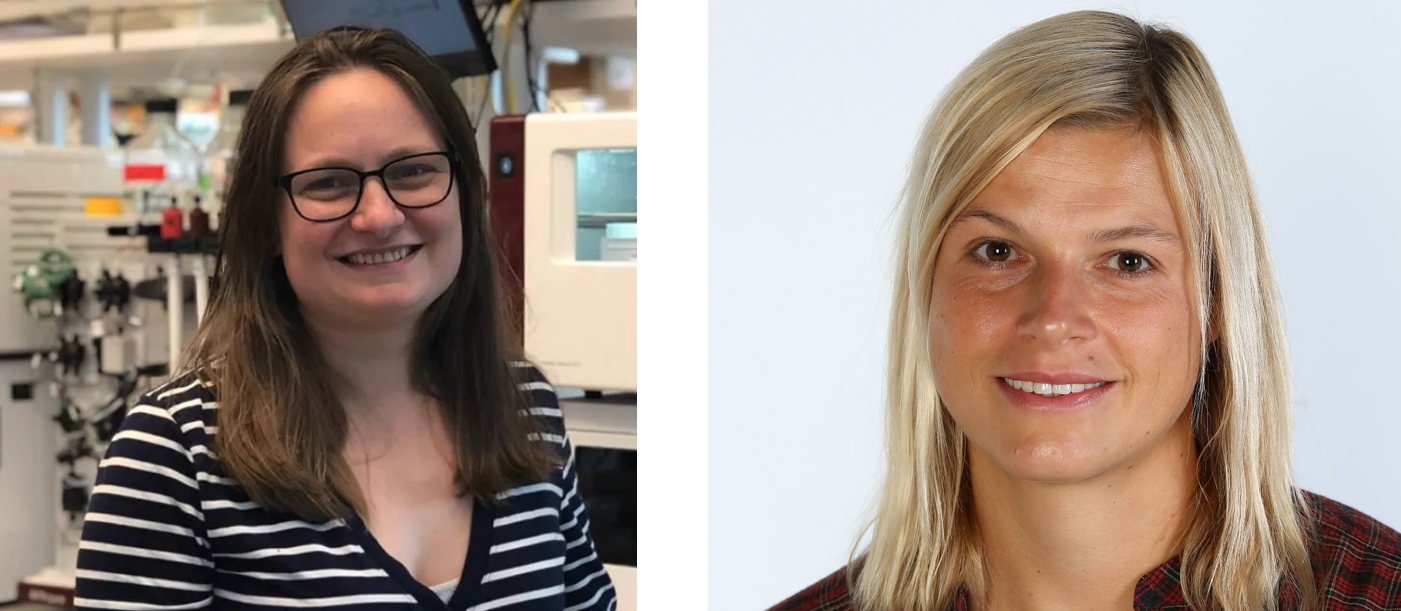
Designing proteins
Parts of our DNA – genes – are transcribed into RNA and then translated into proteins. These proteins are molecules that perform a wide variety of functions in our cells. Among those functions, we find transport. Some proteins carry other molecules while others form ‘tunnels’ through cell walls to ensure that the right things can move in and out of cells. A family of those proteins are known as transmembrane beta-barrels.
Anastassia Vorobieva and her team design such transmembrane proteins to make pores that can act as biological sensors and channels. By combining computation design and experimental characterization, Anastassia and her colleagues recently designed nanopores from scratch. Think of nanopores as single-molecule detectors that can, for example, rapidly sequence DNA. Designing these pores in different sizes and shapes is the first step to building a new generation of synthetic biology tools in the form of biological sensors, scaffolds, and sequencers.
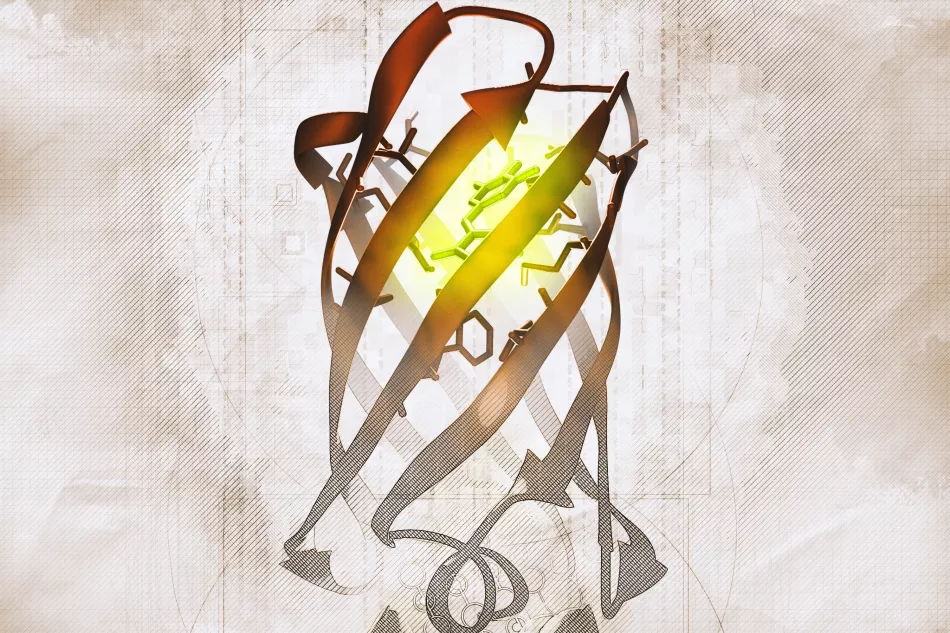
MAGESTIC microbes
Designing genes and proteins is a great start, but we also need to understand how these ‘designs’ are expressed in real life. In other words, to make functional changes in biological systems, we have to chart the paths from genotype (the full genetic makeup of ann organism) to phenotype (all the expressed traits of an organism).
To do so, the lab of Sibylle Vonesch uses MAGESTIC, or Multiplexed Accurate Genome Editing with Short, Trackable, Integrated Cellular barcodes – one of several CRISPR-based approaches for high-throughput genomics. With this method, scientists can engineer thousands of specific mutations in parallel in large groups of yeast cells. Because the mutations have a DNA ‘barcode’, the researchers can observe and quantify which mutations have which effects, as well as how different environments might affect the link between mutation and effect. MAGESTIC effectively proves a tool to generate and screen many yeast mutants that may have industrial and medical applications.

Protein tunnels and MAGESTIC tools are only glimpses of the present and future of synthetic biology. VIB's Tech Watch Core, whose Technology Expert Toon Swings also sits on the organizing committee of the Next-Generation Synthetic Biology (5th edition) conference, has already funded various synthetic biology projects.
As our knowledge of life and our technologies improve, so does our ability to (re)design biological systems. With this power, of course, comes an equally great responsibility to do so ethically.
The potential of synthetic biology is immense. Let’s use it well.
Want more synthetic biology? Grab the final seats at the Next-Generation Synthetic Biology (5th edition) in Ghent, from December 10 to 11. Experts from academia and industry will be there to talk about engineering living systems, from molecules to ecosystems.